Whole-Cell Voltage-Clamp in 350µm Mouse Coronal Brain Slices
- Ian Davies
- Jul 29, 2021
- 4 min read
Abstract
(from Npas4 regulates IQSEC3 expression in hippocampal somatostatin interneurons to mediate anxiety-like behavior, Kim et al, 2021)
Activity-dependent GABAergic synapse plasticity is important for normal brain functions, but the underlyingmolecular mechanisms remain incompletely understood. Here, we show that Npas4 (neuronal PAS-domainprotein 4) transcriptionally regulates the expression of IQSEC3, a GABAergic synapse-specific guaninenucleotide-exchange factor for ADP-ribosylation factor (ARF-GEF) that directly interacts with gephyrin.Neuronal activation by an enriched environment induces Npas4-mediated upregulation of IQSEC3 proteinspecifically in CA1stratum orienslayer somatostatin (SST)-expressing GABAergic interneurons. SST+inter-neuron-specific knockout (KO) of Npas4 compromises synaptic transmission in these GABAergic interneu-rons, increases neuronal activity in CA1 pyramidal neurons, and reduces anxiety behavior, all of which arenormalized by the expression of wild-type IQSEC3, but not a dominant-negative ARF-GEF-inactive mutant,in SST+interneurons ofNpas4-KO mice. Our results suggest that IQSEC3 is a key GABAergic synapsecomponent that is directed by Npas4 and ARF activity, specifically in SST+interneurons, to orchestrate exci-tation-to-inhibition balance and control anxiety-like behavior.
Method
1. Hippocampal CA1 pyramidal neuron electrophysiology
Electrophysiological recordings in brain slices were obtained as previously described (Um et al., 2014). Briefly, P28–34 mouse brains were removed and immediately immersed for 2 min in ice-cold slicing solution (85 mM NaCl, 75 mM sucrose, 25 mM glucose, 24 mM NaHCO3, 1.25 mM NaH2PO4, 2.5 mM KCl, 4 mM MgCl2, and 0.5 mM CaCl2) saturated with 95% O2/5% CO2. Chilled brains were trimmed coronally with razor blades, bonded to a metal platform after dissecting out the olfactory bulb and cerebellum, and then placed in the chamber of a vibratome (Campden 7000smz) filled with ice-cold slicing solution. Coronal sections (0.35 mm thick) were prepared and transferred to a chamber containing artificial cerebrospinal fluid (ACSF; 126 mM NaCl, 2.5 mM KCl, 1.25 mM NaH2PO4, 26 mM NaHCO3, 10 mM glucose, 2 mM CaCl2, and 2 mM MgCl2) for recovery, then initially incubated at 32°C for 30 min followed by incubation at room temperature for 1 h. For whole-cell voltage-clamp recordings in acute cortical slices, patch pipettes (4–8 MΩ) were filled with a 290-mOsm internal solution with the composition, 130 mM Cs-gluconate, 6 mM CsCl, 10 mM HEPES (pH 7.2), 1 mM EGTA, 2.5 mM MgCl2, 2 mM Mg-ATP, 0.5 mM Na-GTP, and 10 mM Na-phosphocreatine. All recordings were performed in ACSF at 28–30°C under a fluorescence microscope (BX50-WI, Olympus). mEPSC and mIPSC recordings were performed with voltage clamped at −60 (reversal potential of GABAA receptor) or 0 mV (reversal potential of AMPA receptor) in the presence of 1 μM tetrodotoxin (TTX), included to block action potential-evoked responses. Membrane statistics in each experiment were monitored by collecting three 1-min traces with a −2 mV voltage step between each trace. Paired-pulse ratios (PPRs) of IPSCs were assessed at 50-ms inter-stimulus intervals. Evoked IPSCs were recorded in the presence of 10 μM 6,7-dinitroquinoxaline-2,3-dione (DNQX; Abcam) and 25 μM AP5 (Abcam). For all whole-cell recordings, membrane statistics were monitored after each trace. Cells for whole-cell recording were rejected if Ra was ≥ 25 MΩ, or if Ra or Rm changed by 25% or more over the course of the experiment. All recordings were digitized at 10 kHz and filtered at 2 kHz. Recordings were monitored with an EPC10 double USB patch-clamp amplifier (HEKA) and analyzed offline using the Mini Analysis program (Synaptosoft).
2. Hippocampal CA1 somatostatin interneuron electrophysiology
Whole-cell voltage-clamp recordings were obtained from acute brain slices. Brain slices were transferred to a recording chamber and perfused with a bath solution of aerated (O2 95%/CO2 5% mixed gas) artificial cerebrospinal fluid (aCSF) consisting of 124 mM NaCl, 3.3 mM KCl, 1.3 mM NaH2PO4, 26 mM NaHCO3, 11 mM D-glucose, 2.5 mM CaCl2, and 1.5 mM MgCl2 at 28–30°C. For measuring postsynaptic currents, patch pipettes (open pipette resistance, 3–5 MΩ) were filled with an internal solution consisting of 145 mM CsCl, 5 mM NaCl, 10 mM HEPES, 10 mM EGTA, 4 mM Mg-ATP, and 0.3 mM Na-GTP. Whole-cell recordings of mIPSCs were performed on CA1 stratum oriens SST+ interneurons, voltage clamped at −70 mV, and currents were pharmacologically isolated by bath application of 50 μM DL-APV (Sigma), 10 μM CNQX (Sigma), and 1 μM TTX (Tocris). Whole-cell recordings of mEPSCs were performed on CA1 stratum oriens SST+ interneurons, voltage clamped at −70 mV, and currents were pharmacologically isolated by bath application of 50 μM picrotoxin (Tocris) and 1 μM TTX (Tocris). For measuring action potential firing, patch pipettes were filled with an internal solution consisting of 130 mM K-gluconate, 20 mM KCl, 0.2 mM EGTA, 10 mM HEPES, 4 mM Mg-ATP, 0.3 mM Na-GTP, 10 mM disodium phosphocreatine. Action potentials fired in 10 s intervals during incremental injection of current (−50 pA to 200 pA). For DREADD validation experiments, 10 μM CNO was bath-applied, after which 1 s, 200-pA current injections were delivered into CA1 stratum oriens SST+ interneurons and action potential firing was recorded. Electrophysiological data were acquired using pCLAMP software and a MultiClamp 700B (Axon Instruments), and were digitized using an Axon DigiData 1550B data acquisition board (Axon Instruments). Data were sampled at 10 kHz and filtered at 4 kHz. Data were discarded if the series resistance was greater than 30 MΩ, or the series resistance differed by more than 20%.
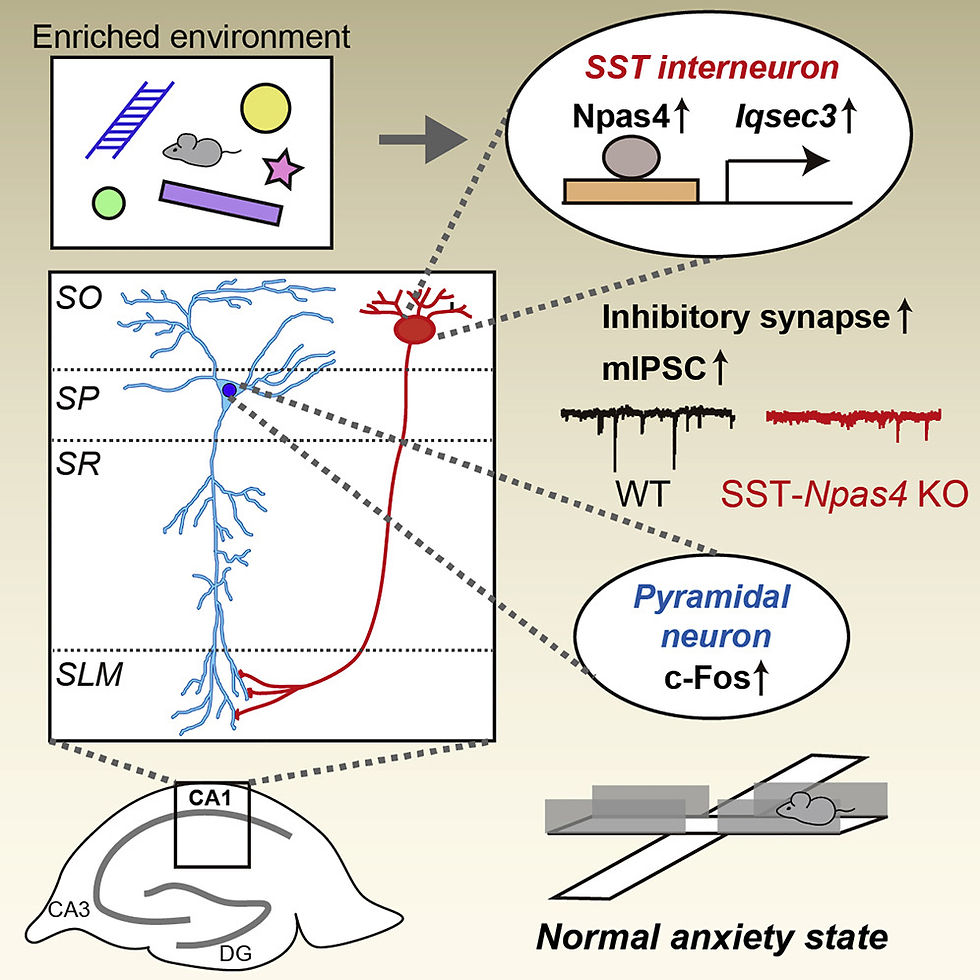
Comentários